Like inland mineral waters, seawater has long been credited with miraculous curative powers. Kiel researchers are putting this belief onto a scientific footing.
Ocean life has the same basic components as life on land. Creatures there have immune systems, they digest food and they fight disease. But the unique nature of the marine environment means that it contains chemicals that are unknown on land, and which can be used to relieve pain or fight disease.
While about 30,000 of these marine natural substances are known to science, the process of getting to an effective and safe compound that solves an actual human problem is a long one. Kiel researchers are working to ensure that these active ingredients are used to best advantage to meet human need for medicine, food and other essentials.
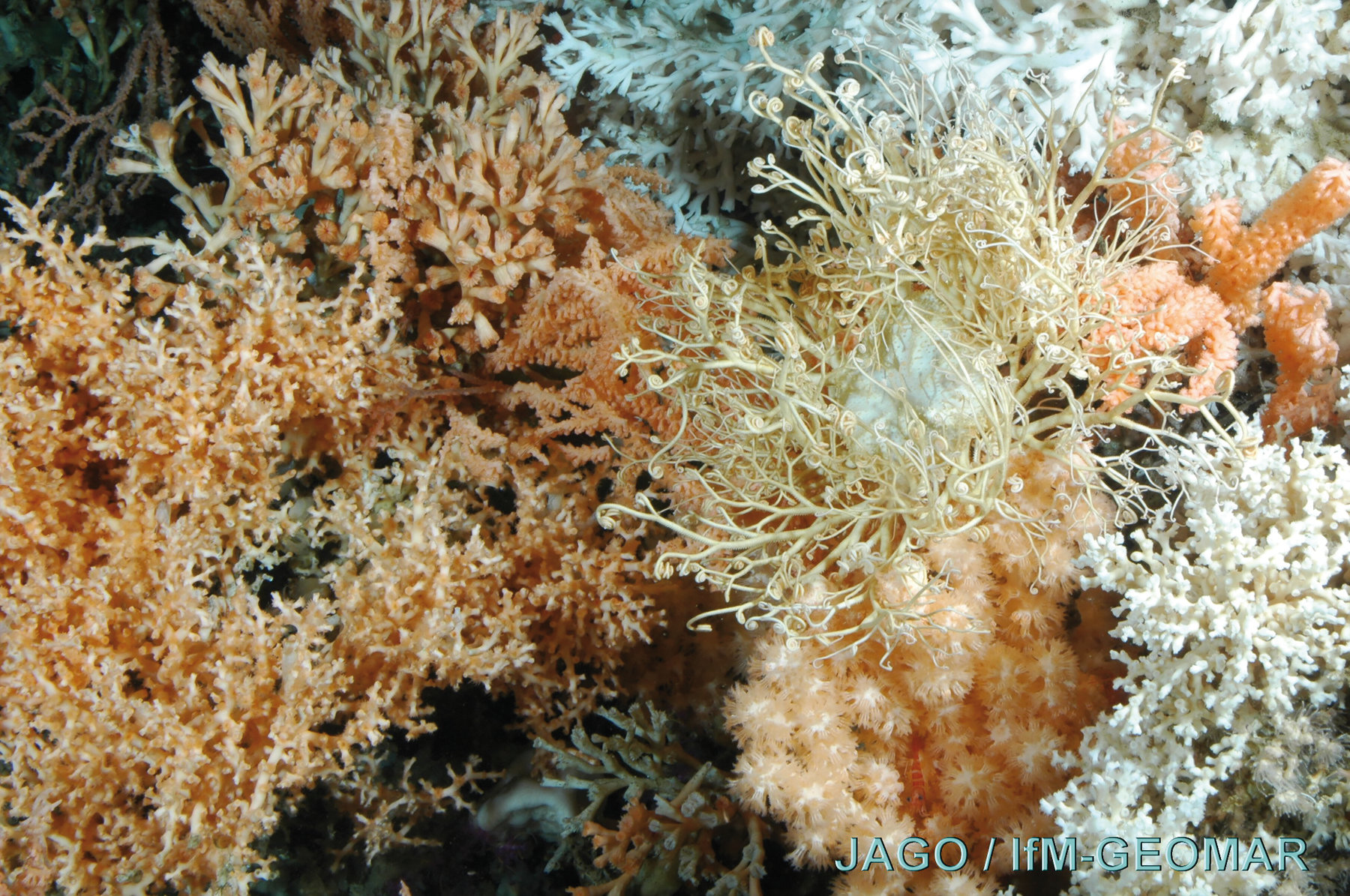
Life began in the ocean and has been evolving there for billions of years. Kiel Research indicates that marine species may become the source of drugs against pain, infection, and diseases including cancer.
Examples include two compounds termed nucleosides, chemical relatives of RNA and DNA. They are derived from a sponge found in the Caribbean, and are the first marine metabolites to be developed into an anticancer drug derived from the ocean. They are also the first ocean chemicals to serve as the scaffold for the development of drugs against diseases, from Herpes simplex to HIV. Other medicines that originate from marine organisms have been obtained from ascidians, molluscs and bryozoans, invertebrate animals that live in mossy-looking colonies.
One problem with this research is that a chemical compound with a novel effect can seem to come from some large invertebrate, when it is in fact made by a much smaller organism living symbiotically in or on its host organism. Discovering exactly where a compound comes from and how it works requires both fieldwork and laboratory science.
While a specific nation owns any living marine resource within its jurisdiction, those found beyond territorial waters are regarded as freely available for use. But Nele Matz-Lück, a maritime law specialist who is speaker for the Kiel Cluster, points out that new proposals being prepared for consideration by the UN General Assembly may alter all this. She says: “The law could change so that these resources are regarded as the ‘Common Heritage of Mankind.’ That could mean creating a licensing scheme for access to genetic resources in areas beyond national jurisdiction, and an accompanying benefit-sharing mechanism.”
Kiel researchers are working with business, government and non-profit bodies to ensure that their discoveries turn into useful drugs and into other types of innovative compound.
How are marine species connected to human well-being? What can we learn from the immune systems of simple marine organisms? Can substances produced by marine animals or microbes cure illness? How do microbes communicate, and what happens if we disturb their communication? What genes can be found in sea life and used by humans?
From ocean mud to coral reefs, the seas are full of chemicals with the potential to cure disease, improve food production and clean the environment.
Why we need microflora
Philip Rosenstiel is a medical doctor. He works on interactions between the environment and mucous membranes in the human intestine. And his research depends on ocean resources.
Any natural environment contains hostile pathogens. Skin and other tissues form biological barriers against them. But in addition, these surfaces are the ecological niche for microflora that carry out essential physiological functions for the host. In humans, these barrier organs can be affected by chronic inflammatory disorders including asthma, inflammatory bowel disease and psoriasis.
“When we study intestinal inflammation, the human host and its associated gut microbiome must be regarded as a single functional unit. Disease mechanisms can only be described if we take both sides of this ‘metaorganism’ into account,” says Rosenstiel. “To answer our research questions, we try to understand regulatory events and bacterial responses in model systems.” And these model systems are marine organisms. They are used in a translational approach to understanding the general principles of human health related to immunology and the maintenance of resident microbial diversity.
Rosenstiel has investigated how these interactions between host and symbiont take place, and how the hosts are colonised [2]. He looks at the symbiosis between the bioluminescent bacterium Vibrio fischeri and its squid host. The squid’s cells regulate enzyme levels to support colonisation by the bacteria. As few as three to five V. fischeri cells (named after Bernhard Fischer, a professor at Kiel in the 19th century) are sufficient to induce a genetic signal within thousands of cells in the squid’s light-organ tissues.
Learning how these interactions between animals and their symbionts work, and how deeply these different species depend upon each other, will help us to understand the inflammatory diseases which result from an imbalanced microbial system within the human body.
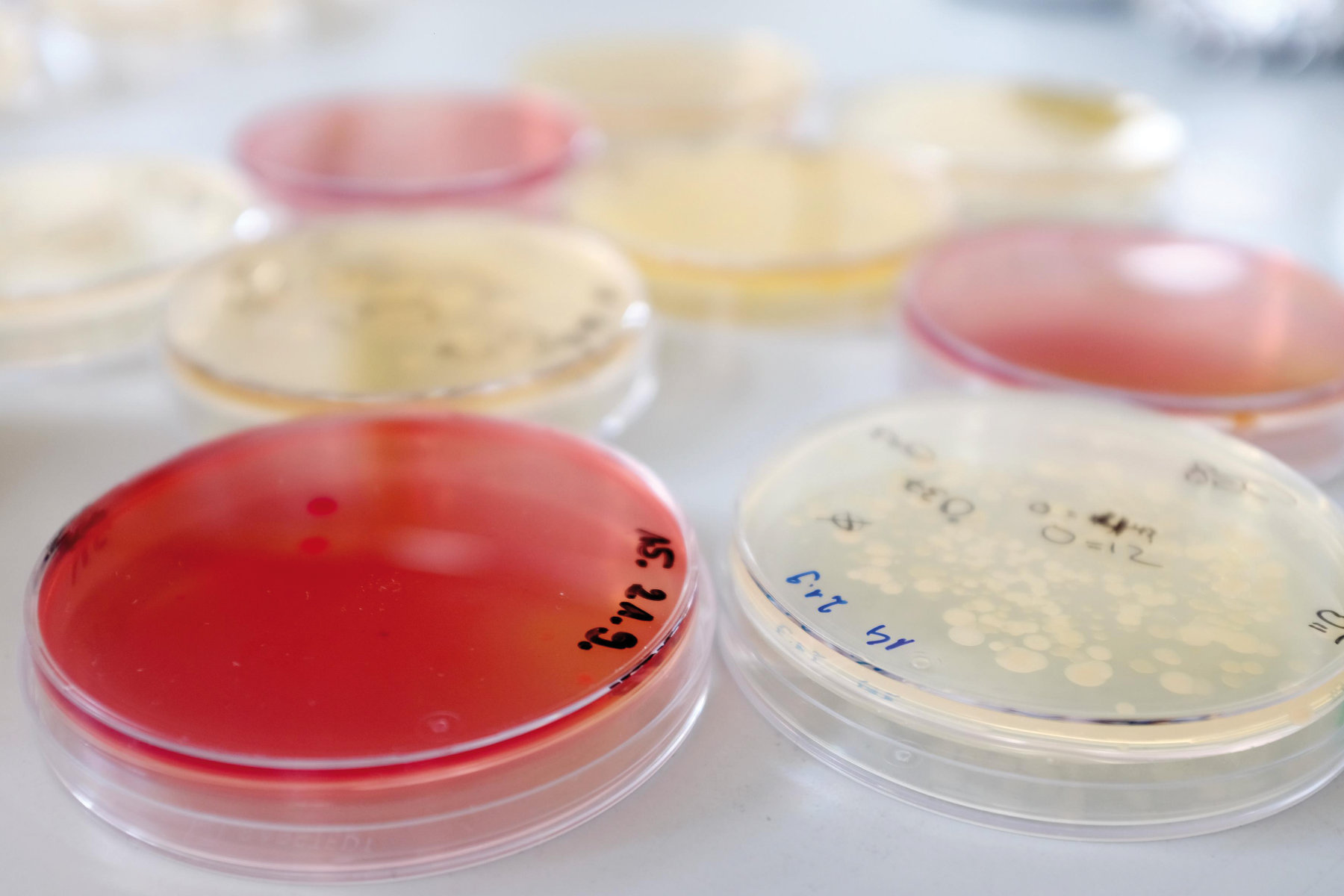
Search for new drugs – a combined effort
A significant new drug would be the most impactful result of Kiel’s work on the ocean’s genetic resources. But only seven major drugs have been developed from ocean resources in 40 years of intensive drug discovery efforts around the world. The process of isolating a few milligrams of the compound to be scaled up to enter clinical trials, and then patenting and licensing it for medical use, is an exceptionally expensive, risky and time-consuming one, involving a minimum of 10-15 years of effort.
However, there are other product areas in which specifications are less demanding and legislative requirements are simpler to satisfy. Kiel marine science is active in many of them. They include foods for people and for animals, agrochemicals, fuels and cosmetics. In some cases, it may not even be necessary to extract the active ingredient from the source organism. It is possible to add marine microorganism to soil in order to kill soil-borne crop pathogens.
Another possible product is what Tasdemir terms “probiotics for fish.” At the moment, fish farms have a big impact on the coastal marine environment, because of the large amounts of antibiotics they use to keep fish healthy. Kiel research (page 56) has shown that this limits the growth of the aquaculture industry. New and more natural probiotics are being developed which would strengthen the immune systems of the farmed fish and keep them free from infection, while reducing impacts on other marine life.
This work is being carried out through the mentoring of a Latvian company, Baltic Probiotics, under the umbrella of the Baltic Blue Biotechnology Alliance project, led by Tasdemir [3]. This grouping of over 25 businesses and research organisations aims to exploit the massive potential of the Baltic Sea to supply novel, sustainable biotechnology products for the benefit of the region and the rest of the world.
Tasdemir says: “We are using this initiative to develop awareness in industry, and among the public and politicians, of the potential of marine biotechnology. We approach small enterprises, or even academics with business minds, and find out their problems in product development, all the way from research to business plan. How do they identify business needs and get the expertise they need? At Kiel, we plan to build a database of experts and expertise that can help. If it works, this approach can be replicated in other regions.”
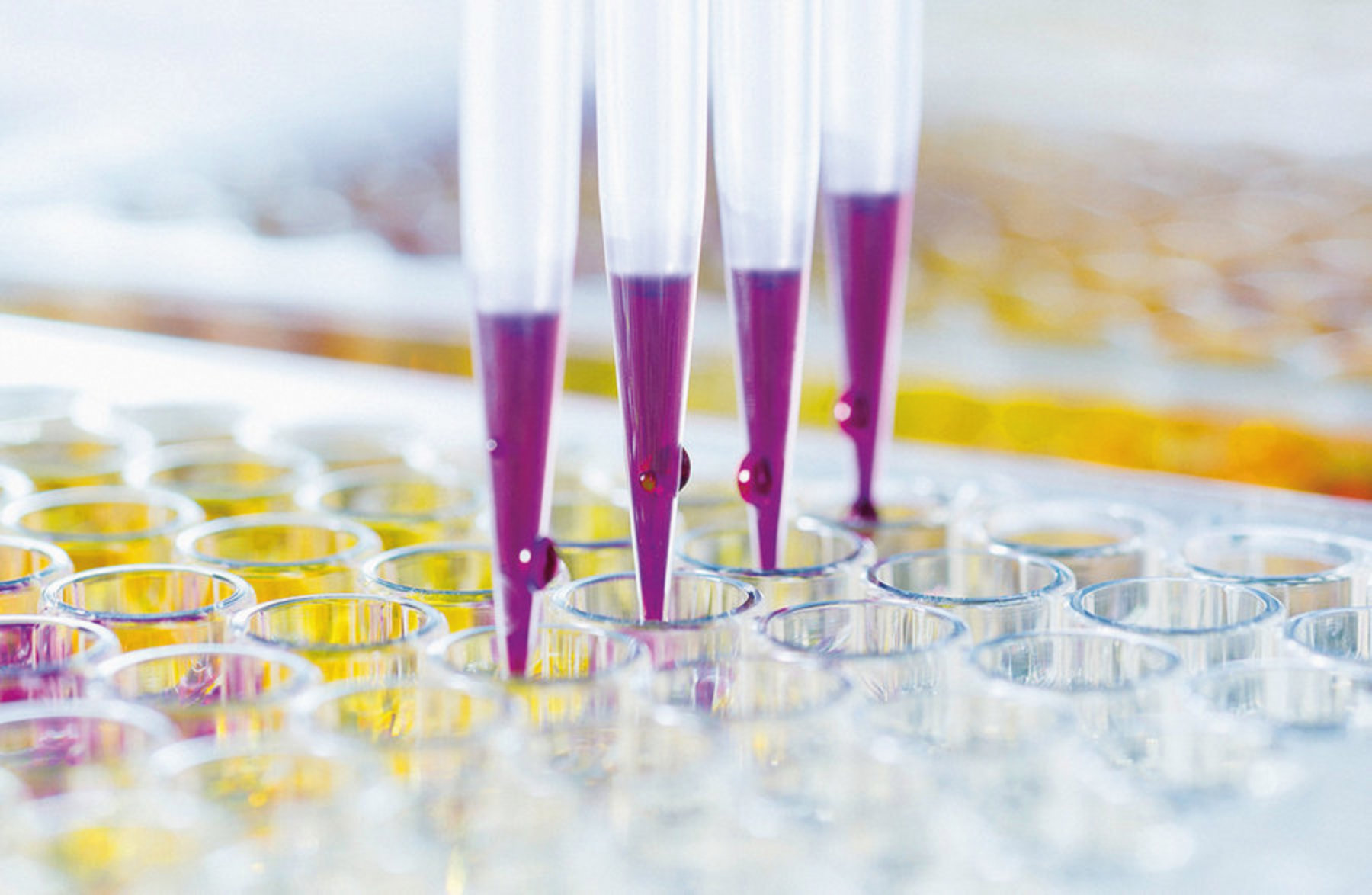
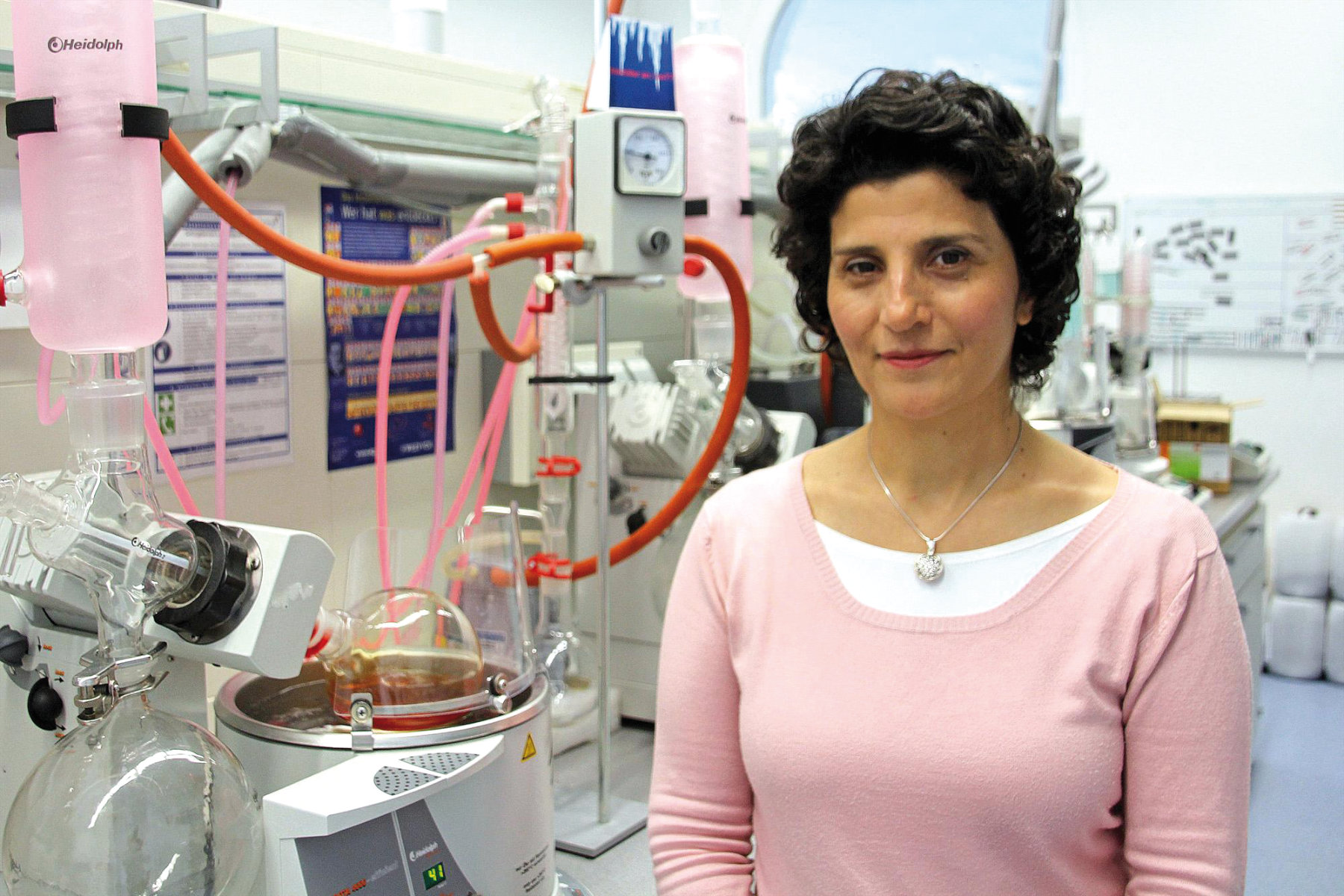
Marine drugs?
Most people would find the view from Deniz Tasdemir’s office all too distracting. From a building near the mouth of the Kiel Canal, she has a constant prospect of ocean-going ships making their way through the world’s busiest inland waterway.
But Tasdemir is not diverted by this prospect. Her focus is on the ocean not as a highway, but as a source of new chemical compounds of benefit to the human race. Her focus is on marine biodiscovery, the main pillar of marine biotechnology. It involves the hunt for exotic chemicals developed by marine organisms through millions of years of evolution in the seas and oceans. And she explains that the implications are massive. Most obvious is the potential for new drugs and medical treatments to emerge from her research, mainly anticancer agents and antibiotics. But in addition, there could be new “nutraceuticals,” foods with added health properties, chemicals for the bioremediation of polluted land and water, and even new cosmetics. Marine-derived antifouling agents might be created as a natural and less polluting way of preventing organisms encrusting ship hulls. This would mean replacing today’s toxic and less effective antifouling agents with natural molecules coming from the sea. Other marine products include an analgesic 1,000 times more powerful than morphine. This small peptide is derived from a snail which uses it to paralyse fish which it then eats.
Tasdemir points out that most medicines, including familiar ones such as aspirin, come from nature. They may be natural products, or synthetic ones derived from them. She adds: “So far, most drugs have been derived from terrestrial plants. But life began in the sea and has longer to evolve there.” [1] In addition, the ocean contains many extreme environments. To survive there, organisms need to innovate. Tasdemir says: “In the deep ocean or the polar regions it is cold and dark. There are low nutrient levels and extreme pressures. So anything living there needs a good strategy to survive. The biosynthesis of unusual chemical compounds for adaptation and survival is a known mechanism in extreme environments. This means that many exotic compounds can be isolated from so-called extremophilic organisms.”
While drug discovery normally involves small molecules, larger ones such as cold- or heat-adapted enzymes are of interest to the food industry and for other applications. The texture of some well-known ice-creams depends upon the way they do not freeze solid even below zero degrees Celsius. This is because they contain a natural antifreeze derived from a deep-ocean fish.
However, most of the compounds studied in Tasdemir’s laboratory are derived not from these advanced animals but from microbes. One millilitre of seawater contains a million microbes, while the same amount of sediment contains a billion. These latter are of interest because most of the antibiotics we use today come from sediment bacteria. At the same time many larger sea animals, such as sponges, contain a complex array of symbiotic bacteria and fungi that produce food or other useful chemicals for the host. Sponges do not have any internal organs. Instead, about half their body weight is made up of microbes with their own special functions and a highly evolved genetic structure. “Most of the molecules we obtain from marine animals are in fact produced by these microbes,” she says.
War zone
Tasdemir says that once microorganisms are isolated from sediment or from their animal hosts, it is important not to let them get too comfortable. If you put them in a Petri dish and feed them with artificial nutrients, they have no need to produce novel chemical compounds. In nature, all these microorganisms are in harsh competition for nutrients or other resources. Some produce interesting molecules to communicate, fight and deal with environmental stresses or cues. In the lab environment, microbes are subject to none of these stimuli. So she is now putting two or more microbe species from the same environment together.
Tasdemir says: “If you put rival species together, you get competition. They switch on chemical pathways that produce compounds that have a role in attack or defence. That cell-to-cell communication creates a ‘war zone’ with a rich chemistry of toxins, and often creates antibiotic activity that we can exploit. Seeing these pathways in action can give the biotechnology industry the strategies it needs to produce novel compounds.”
Despite their beauty, this competition is seen at its most savage in the world of the coral reef. Here creatures are forced to compete and interact in a tiny space. To do this, they create a vast range of defensive and offensive chemicals. These molecules are often toxic and can have biological properties such as anticancer activity. In addition, much of the action on a coral reef takes place in shallow water which receives a lot of sunlight. So the animals here have evolved their own sun protection strategy. One commercial sunblock cream makes use of a natural compound evolved on the Great Barrier Reef in Australia.
And while the genetic diversity of reef creatures has long been recognised, we are now becoming more aware of the biological potential of the sea floor and the sediments that underlie it. This material can be harvested by remotely-operated vehicles or by coring machines.
But Tasdemir stresses that developing a useful product from a promising chemical in the ocean, or in a laboratory dish, is a long and complex process. The so-called supply issue is the main bottleneck. To avoid harming the natural ecosystem, we collect only small amounts of any organism. This means that only a few milligrams of the sought-after compound becomes available for all the chemistry and pharmacology work that is needed. And even then, she says: “The second hard part is to characterise the very complex structure of the compound and its mode of pharmacological action. After that, synthesising it is less of an issue.” Fortunately, new technology is shortening timescales. “Computational chemistry is speeding up the process of determining the structure and action of these compounds. This is essential because industry is only interested in agents whose structure and action are known and which we are sure have low toxicity in humans.” There is no point in a cure for cancer that kills the patient.
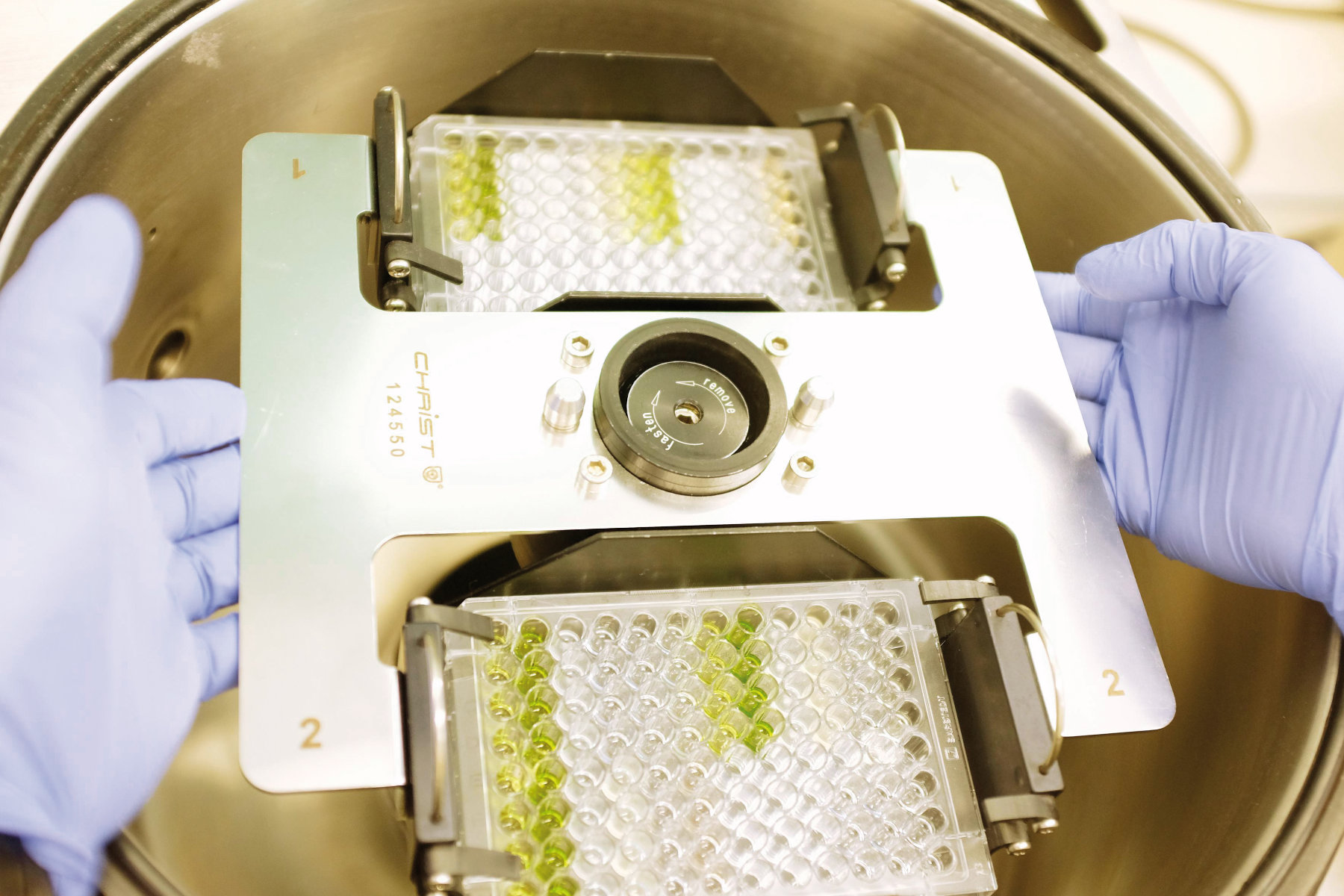
Biofilms
If you have had to clean that horrible black mess that builds up around the detergent tray in the washing machine, you already know about biofilms. These are masses of living cells attached to a natural or artificial surface, anything from the top layer of the ocean to the inside of the human body.
Biofilms are especially dangerous when they form on medical equipment or implants. The infectious cells they contain are impossible to destroy. The best approach is to stop them forming in the first place, and scientists from Kiel and Hamburg Universities are developing ways to do this.
Professor Ruth Schmitz-Streit, from the Institute of General Microbiology, says that they are looking at this problem through the lens of basic science. In order to establish themselves on surfaces, the bacteria in a biofilm must communicate with each other via signal molecules. If this communication is disrupted, no biofilm can be formed. And this cell-to-cell communication can be disrupted by proteins which break down these signal molecules or make them ineffective [4].
The researchers have concentrated their search for these proteins on natural environments outside the laboratory. They took samples from seawater, from glaciers, from jellyfish, and indeed from the biofilm residue in a washing machine. They extracted DNA from the samples, and used it to identify proteins with the ability to break down the signal molecules or render them ineffective.
They found that the marine environment contains many proteins which can prevent cell-to-cell communication. “As the oldest ecosystem, the marine setting is incredibly rich in new, undiscovered substances. It offers huge potential for these biologically active proteins,” says Schmitz-Streit. Particularly important was their discovery of the communication-disrupting protein QQ-2. This protein can prevent many different types of bacterial biofilms from forming. Its discovery may lead to the development of medications to fight the growth of disease-bearing biofilms in hospitals and other critical locations.
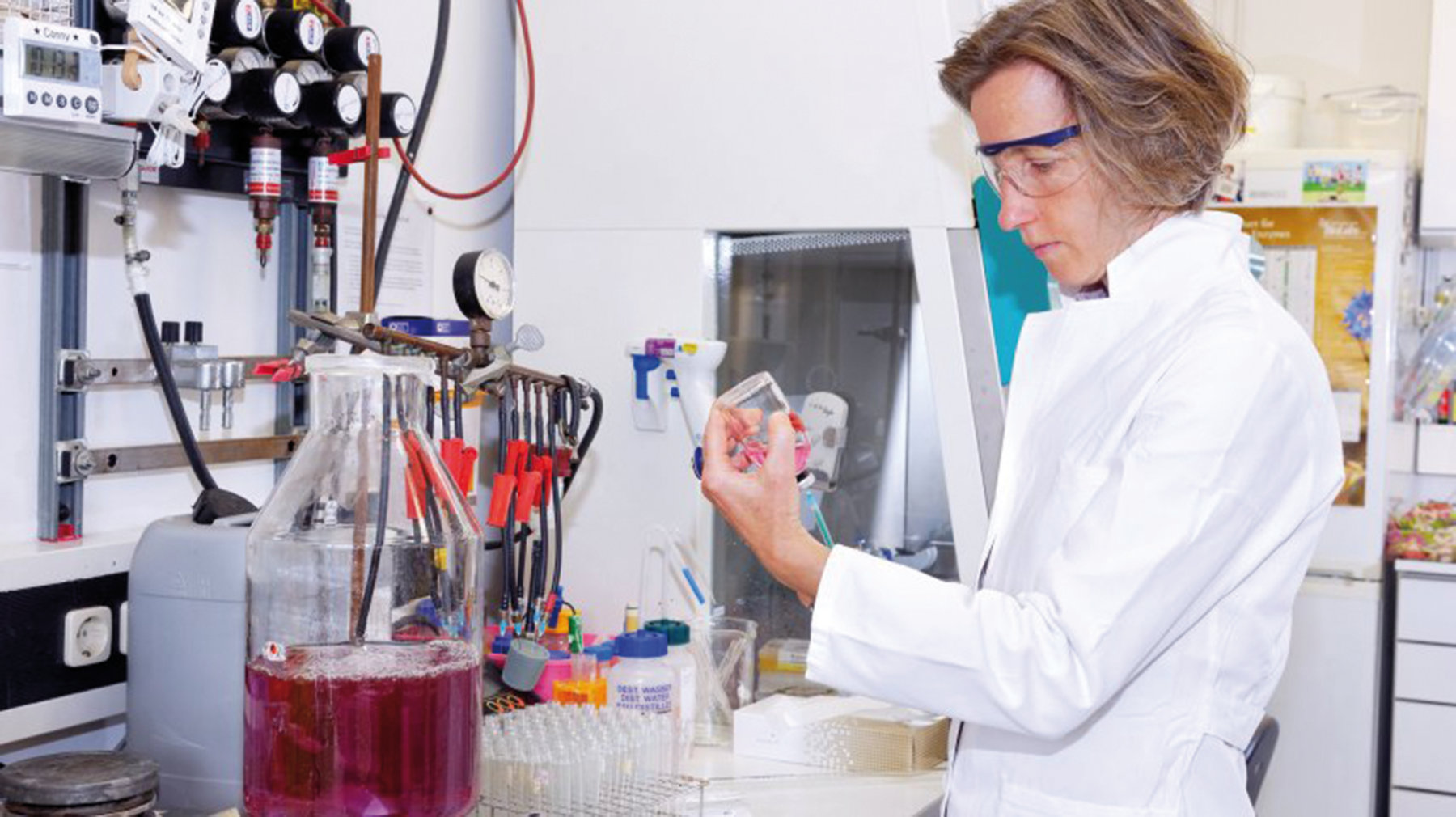
Outlook
We now think that pathogenic microbes and parasites are important regulators of marine populations and biodiversity. This prompts the question: how many pathogens make up a healthy ocean, and what is their role in sustaining a functioning ecosystem? There is mounting evidence that the abundance of marine pathogens is increasing as a result of anthropogenic pressure.
The emergence of novel diseases from the marine realm is well-documented. Examples include the increased incidence of Vibrio species, bacteria that can cause infection in humans. Shellfish toxicity can be associated with harmful algal blooms, and viral diseases of marine origin may have the ability to attain epidemic proportions.
To date, only a minute fraction of the ocean’s microbial richness has been studied. As we carry out a thorough assessment of the services provided for human well-being by ocean microbes, we expect important insights into infection biology to emerge. Kiel research into marine life will tell us more about the potential for harm from these species, so that the risks can be assessed and countermeasures developed.
References
[1] Johanna Silber, Annemarie Kramer, Antje Labes and Deniz Tasdemir (2016) From Discovery to Production: Biotechnology of Marine Fungi for the Production of New Antibiotics, Marine Drugs, 14(7), 137; doi:10.3390/md14070137
[2] Kremer N, Philipp EE, Carpentier MC, Brennan CA, Kraemer L, Altura MA, Augustin R, Häsler R, Heath-Heckman EA, Peyer SM, Schwartzman J, Rader BA, Ruby EG, Rosenstiel P, McFall-Ngai MJ. Initial symbiont contact orchestrates host-organ-wide transcriptional changes that prime tissue colonization. Cell Host Microbe. 2013 Aug 14;14(2):183-94. doi: 10.1016/j.chom.2013.07.006. PubMed PMID: 23954157; PubMed Central PMCID: PMC3928804.
[3] www.balticbluealliance.eu
[4] Weiland-Bräuer, N., Kisch, M. J., Pinnow, N., Liese, A. und Schmitz-Streit, R. A. (2016) Highly Effective Inhibition of Biofilm Formation by the First Metagenome-Derived AI-2 Quenching Enzyme Frontiers in Microbiology, 7 (1098). DOI 10.3389/fmicb.2016.01098.