Most of the Earth's surface is ocean, but the metals we use are almost all mined on dry land. There are abundant minerals on the sea floor, but is it practical to use them? Major environmental and ethical questions arise from exploiting areas whose ecological resilience has not been explored. An interdisciplinary approach is needed to reach valid solutions.
The floor of the deep ocean is a dark and mostly slow-changing environment. But scientists led by Kiel marine researcher Sven Petersen point out that over geological time scales of millions of years, this makes it the perfect site to mine rich mineral deposits.
Several deep-sea minerals have been identified that might prove economically viable to extract [1]. The best-known example are manganese nodules that form on the muddy abyssal plains over millions of years. The nodules are found in old, stable regions of the ocean floor, especially in the Pacific, and typically in water depths of 3000-6000m. Because they form so slowly, they do not occur in geologically active areas where the seafloor is continuously being replaced by new lava. The Kiel experts estimate that manganese nodules might be found in economically viable amounts across more than 50 million square kilometres of the deep ocean. Most of these areas lie beyond areas of national jurisdiction, the so-called Exclusive Economic Zones (EEZ), of any nation. The greatest concentrations of metal-rich nodules occur in the Clarion-Clipperton Zone (CCZ) of the eastern Pacific Ocean, which extends from the west coast of Mexico to Hawaii.
While they consist largely of iron and manganese compounds, these nodules are referred to as “polymetallic” as they host other elements of economic interest such as nickel, copper, cobalt, lithium, and the so-called rare earth elements. Copper and nickel are among the most important metals for today’s human society, especially copper for its pivotal role in electrical equipment. Lithium and cobalt are needed in today’s batteries, while the rare earths are widely used in electronic devices and in renewable energy technology, especially in wind turbines.
Can the ocean supply raw materials for future generations? As land-based mineral resources become increasingly difficult and expensive to acquire, the potential for mining them from the deep sea floor has become more widely discussed and debated. However, the quantity and quality of these seafloor resources are uncertain, as are the risks to deep-sea ecosystems.
Deep-sea mining forms part of the debate on the United Nations Sustainable Development Goals. We need to address the economic, scientific, legal and diplomatic challenges that arise from the possible use of these resources.
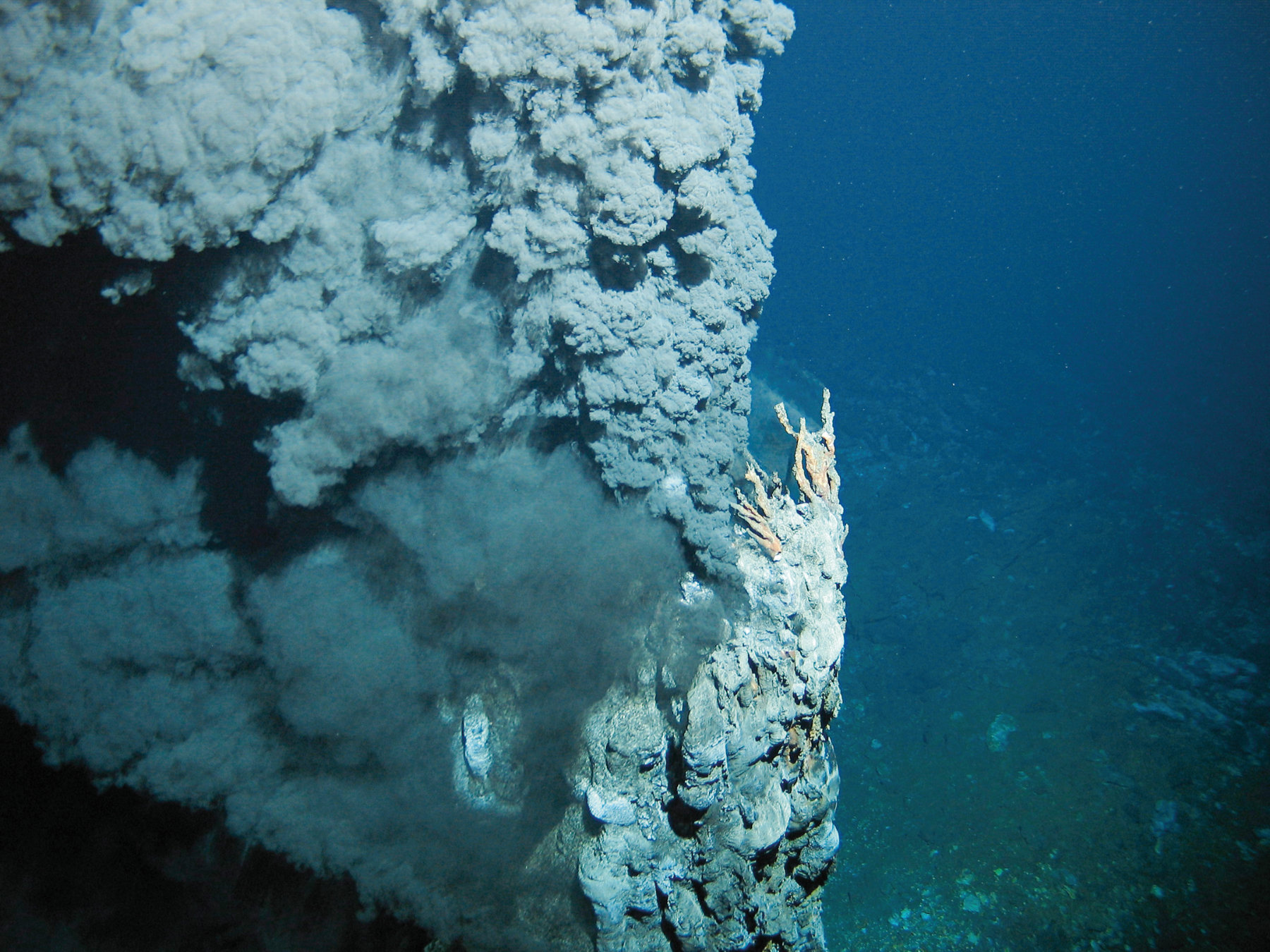
Research in Kiel is contributing to assessments of the volume and metal content of deep sea ores, and of the environmental and legal consequences of their exploitation. This is an exceptional form of interdisciplinary research spanning basic natural sciences, economic models and legal questions.
In theory, the nodules hold vast reserves of accessible metal. The nodules from the CCZ alone are thought to contain three times as much nickel and five times as much cobalt as the current reserves known on land.
In addition, the mining of cobalt-rich manganese crusts that form on the flanks of old volcanic seamounts also seems viable. The most promising region for this kind of deposit lies in the western Pacific. Like the nodules, these crusts contain mainly iron and manganese. But they hold large quantities of cobalt, tellurium and the rare earth element yttrium that far exceed land-based reserves.
Petersen and his co-authors have also examined mineral deposits in the neighbourhood of “black smokers,” hot subsea vents in volcanically active regions of the ocean floor. These deposits are rich in copper, zinc, and in some cases gold and silver. Some occurrences contain other valuable elements such as selenium and cadmium, but may also contain abundant toxic metals such as mercury or arsenic. It is much harder to assess the commercial potential of black smoker deposits. Unlike manganese nodules and crusts, their deposits are three-dimensional, and information on their depth and therefore their size is lacking. The biggest deposits of this kind host a few million tons of ore, which is small compared to land-based deposits.
But further Kiel research suggests that we may be underestimating the scale of these deposits. Jörg Hasenclever and colleagues from GEOMAR, the UK and Norway have examined the basic science of so-called “hydrothermal” zones in the ocean [2]. They find that these regions are the sites of hot-water circulation which can lift metallic elements from deep inside the Earth to an accessible location. Their calculations show that the amounts of metal that could be mobilized by hydrothermal flow are far greater than current estimates based on the deposits we know of today. It is still unclear where these missing metals are.
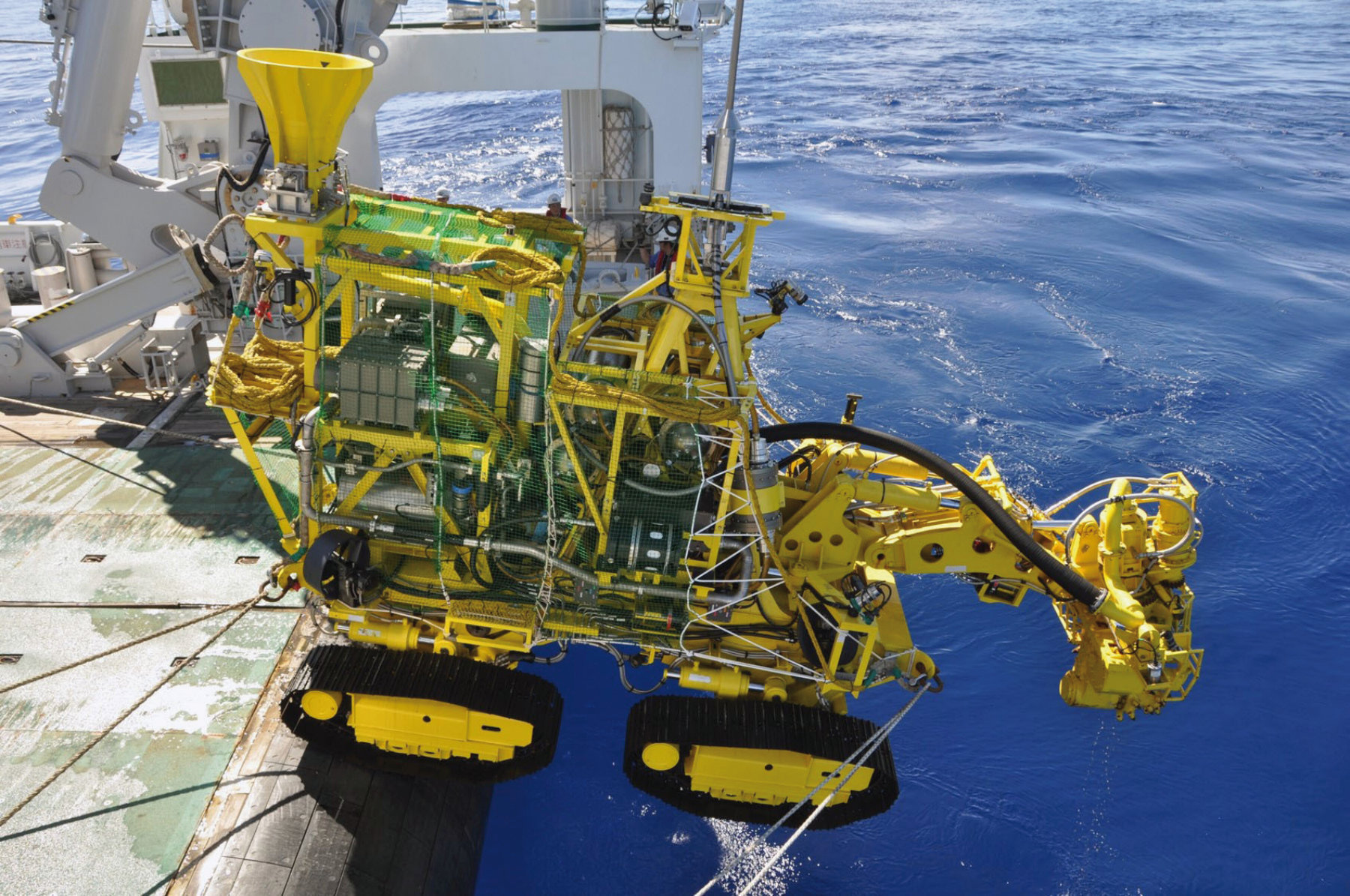
Chance to do it right
Although the decades-long debate about the technological and economic challenges of nodule mining continues, the International Seabed Authority (ISA) has issued 17 licences to allow the exploration of these resources in recent years. Most are for the Clarion-Clipperton Zone in the Pacific. In addition, the Cook Islands have issued licences for nodule exploration in their own waters and covering 100,000 square kilometres of seabed in its EEZ. France, Korea and Japan also have EEZ areas that may be suitable for marine mineral mining in the Pacific, in France’s case via its overseas territories.
Petersen and his fellow experts from Kiel and the United States point out that extracting these resources means significant disturbance of seafloor areas of which we have incomplete knowledge. Mining a million tonnes of metal in the form of manganese nodules would involve disturbing about 75 square kilometres of the seabed. They add that while a typical nodule is 1-5cm in size, the cobalt-rich crusts can be 4-10cm or more in thickness. This means that they can be exploited across smaller areas, perhaps 12 square kilometres for every million tonnes mined.
Nodule mining would involve massive dredges sweeping over huge areas of the deep ocean floor. If this happens, say Petersen and colleagues, “ecosystems in the deep ocean plains may never recover to the original species richness and distribution, due to the substantial loss of hard substrate.” While the resources available at black smokers have yet to be fully defined, it is possible that they would recover more effectively, since they are located in a fast-changing volcanic environment that is renewed far more often than the deep ocean floor.
The authors add that because deep-sea mining has not yet developed, there is a chance to get the legal and environmental regulations right before mining starts. The ISA is responsible for producing the rules that govern this possible future activity, which means that there is space for academics, NGOs and other stakeholders to influence their final form. Nine nodule-rich areas of the seafloor have already been declared Areas of Particular Environmental interest (APEIs) by ISA and are out of bounds for future mining activities. These APEI cover 400x 400km each, totalling 1,440,000 square kilometres of the Pacific. Two additional areas are being considered for this status.
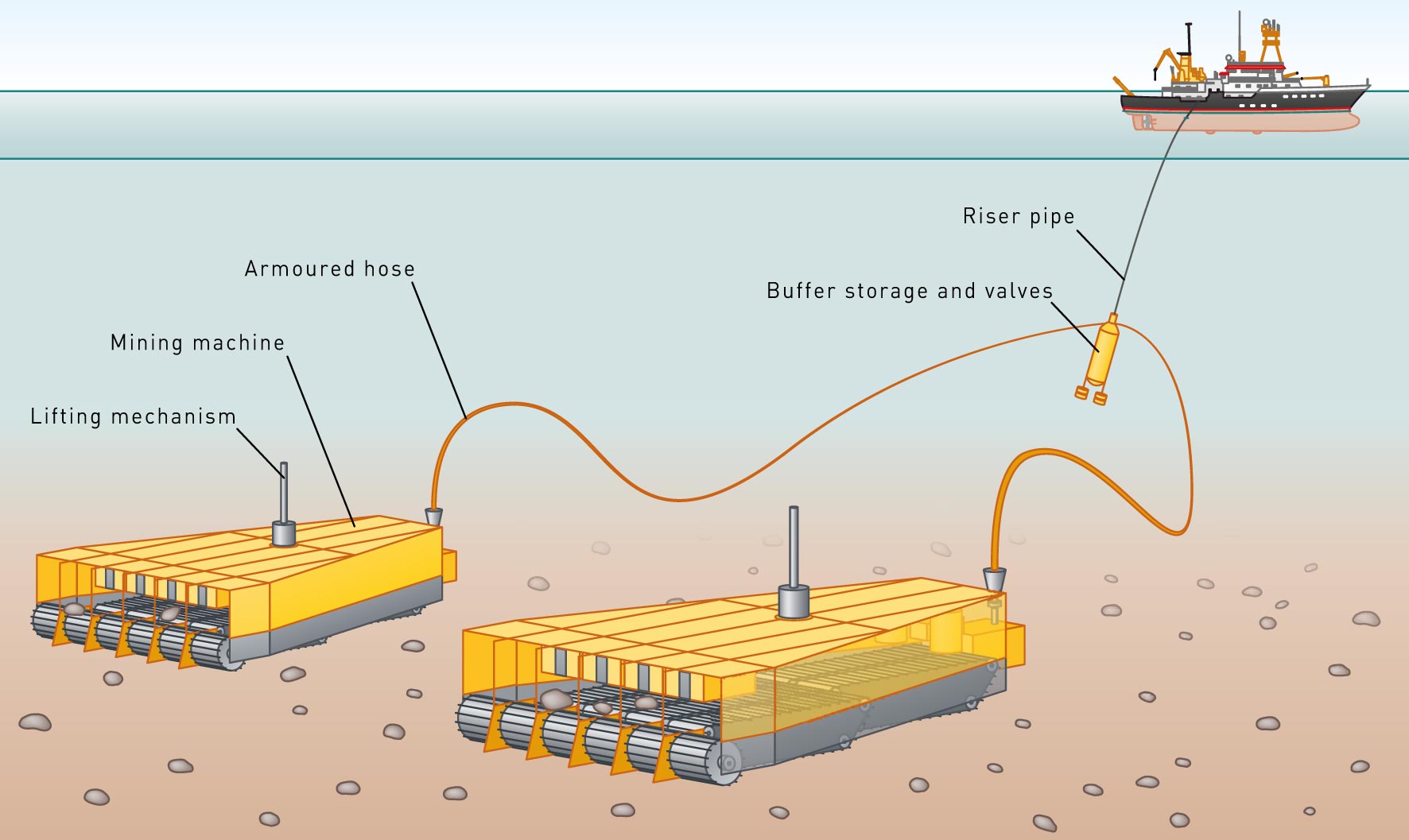
What do we need to learn?
The overall resource potential of deep sea minerals seems immense. But the question of how mankind's need for energy and metals can be met in the future, and whether deep sea resources will play a significant role, cannot be answered at present, due to the lack of information on their distribution and metal content. It is important, however, to provide basic geological information as a basis for political, economic and societal discussions of the prospects for deep-sea mining [3].
The possibility of deep-sea mining implies that exploratory work needs to be carried out in vast areas of the global ocean floor that have never been investigated in detail. This research will need to be complemented by improved frameworks for assessing the societal and environmental impact of marine resource exploitation. The benefits of this new technology depend on the trade-off between the market value of these resources and the risks of their exploitation.
At present it is impossible to assess this trade-off. We lack fundamental knowledge of the implications for biology and oceanography. So we cannot envisage how the value of a marine mineral deposit compares to the risks of mining it. This issue must be addressed by combining different research disciplines, linking natural science with economic studies, legal assessments and the discussion of ethical questions.
It is also important to realise that many geological resources exist close to the shoreline in submerged areas of the continental shelf [4]. Mark Hannington and colleagues suggest that it may be feasible to mine these deposits from a base on land. This approach could minimise environmental risk to seafloor ecosystems while helping to meet global resource demands.
Nele Matz-Lück, a specialist on the international law of the sea and Cluster speaker, says: “It is not yet clear how the law will develop as our knowledge of the deep ocean improves. The international legislation on its exploitation is so far only in draft form and needs to be reassessed as science finds out more. The law needs to be able to deal with uncertain impacts from this new technology, and will have to change in the light of new evidence”.
Gas Hydrates in a warming environment
The gas you probably burn to cook your dinner is methane, produced alongside oil from deep wells running thousands of metres into the Earth’s crust. But there is another type of methane trapped inside the Earth, and Kiel scientists have been looking at the hazards and benefits that go with it.
Called Methane Hydrates, these deposits are a mixture of methane and water found in cold conditions at shallow depths in the Earth, often in lakes or on continental margins.
Because they are only stable in cool conditions, it is possible that warmer ocean temperatures will cause some of these hydrates to decompose. When this occurs, the methane is released into the water above, and potentially into the atmosphere. It seems that this happened during a global warming episode at the start of the Eocene epoch, 56 million years ago. Methane is a greenhouse gas, so releasing it into the atmosphere in large amounts poses the risk of further global warming.
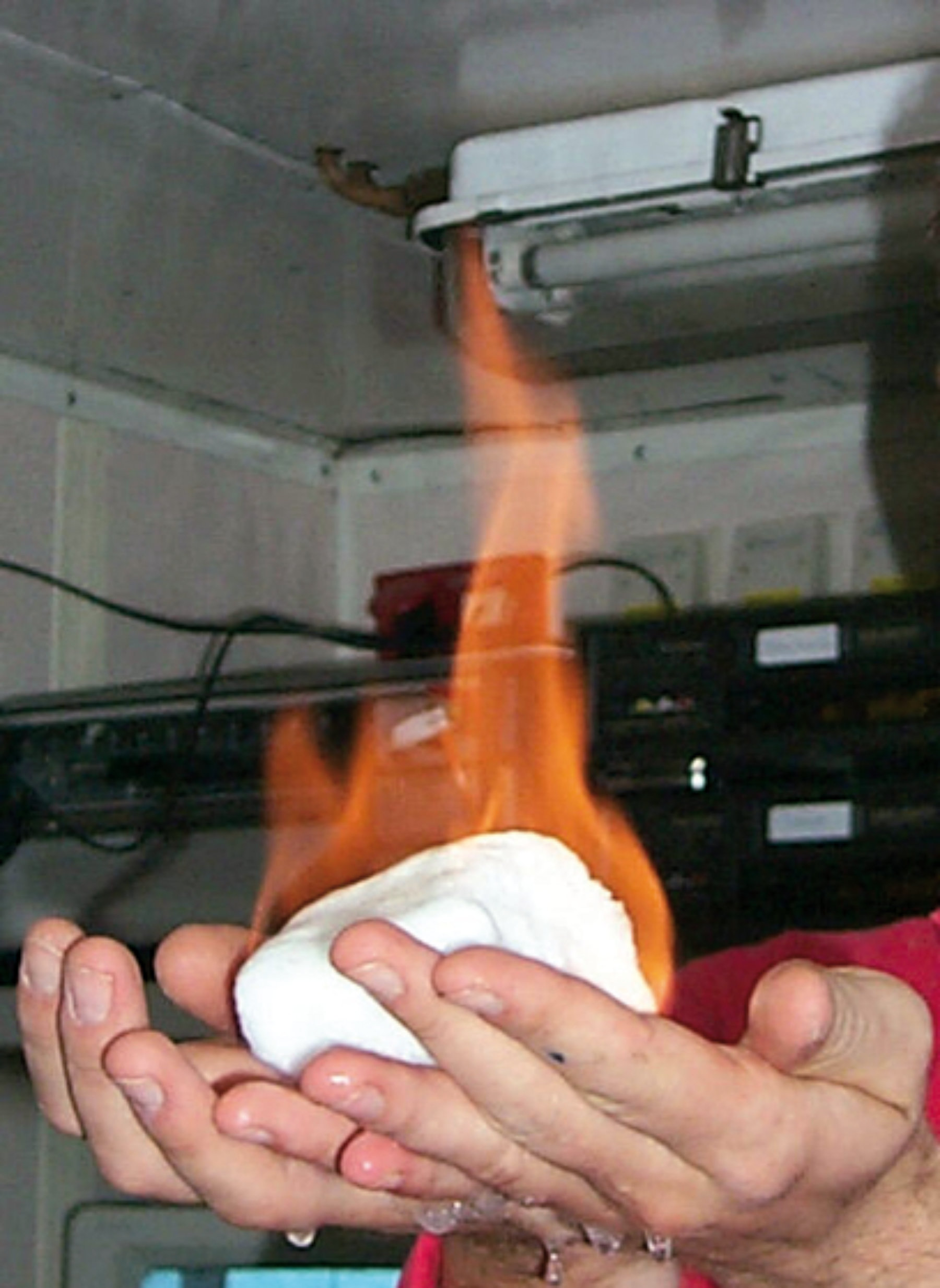
Kerstin Kretschmer and colleagues at Kiel have shown that over 1.1 trillion tonnes of methane are trapped in these hydrates [5]. However, predictions for ocean bottom temperatures over the next century are reassuring. They imply that less than 500 million tonnes of methane will be released, mainly from deposits less than 700m below sea level. This, they say, is “negligible compared to the current anthropogenic releases of methane and other greenhouse gases.” Substantial amounts of methane are also locked up on land, including large volumes in permafrost in the Arctic. The possible release of this methane is a further global warming hazard.
But these findings do not mean that we can forget about methane hydrates. Methane leaks and gas flares have already been observed that are probably caused by these hydrates decomposing.
In addition, they are a usable source of hydrocarbons and can in theory be exploited. Work by Lena-Katharina Doepke and Till Requate at Kiel has pointed out that this exploitation could lead to unintended methane leakage, as well as to extra carbon dioxide emissions when the methane is burned [6]. Both of these would contribute to climate change. More intriguingly, removing these deposits would destabilise geological structures at the continental margins, and so increase the risk of submarine slides.
Doepke and Requate say that new policies are needed to manage this risk. They call for global agreement on a tax regime which would compensate for the pollution due to using methane from hydrates, and for the potential damage due to increased risks of submarine mass wasting. More research is needed to define how big and how frequent these slides would be.
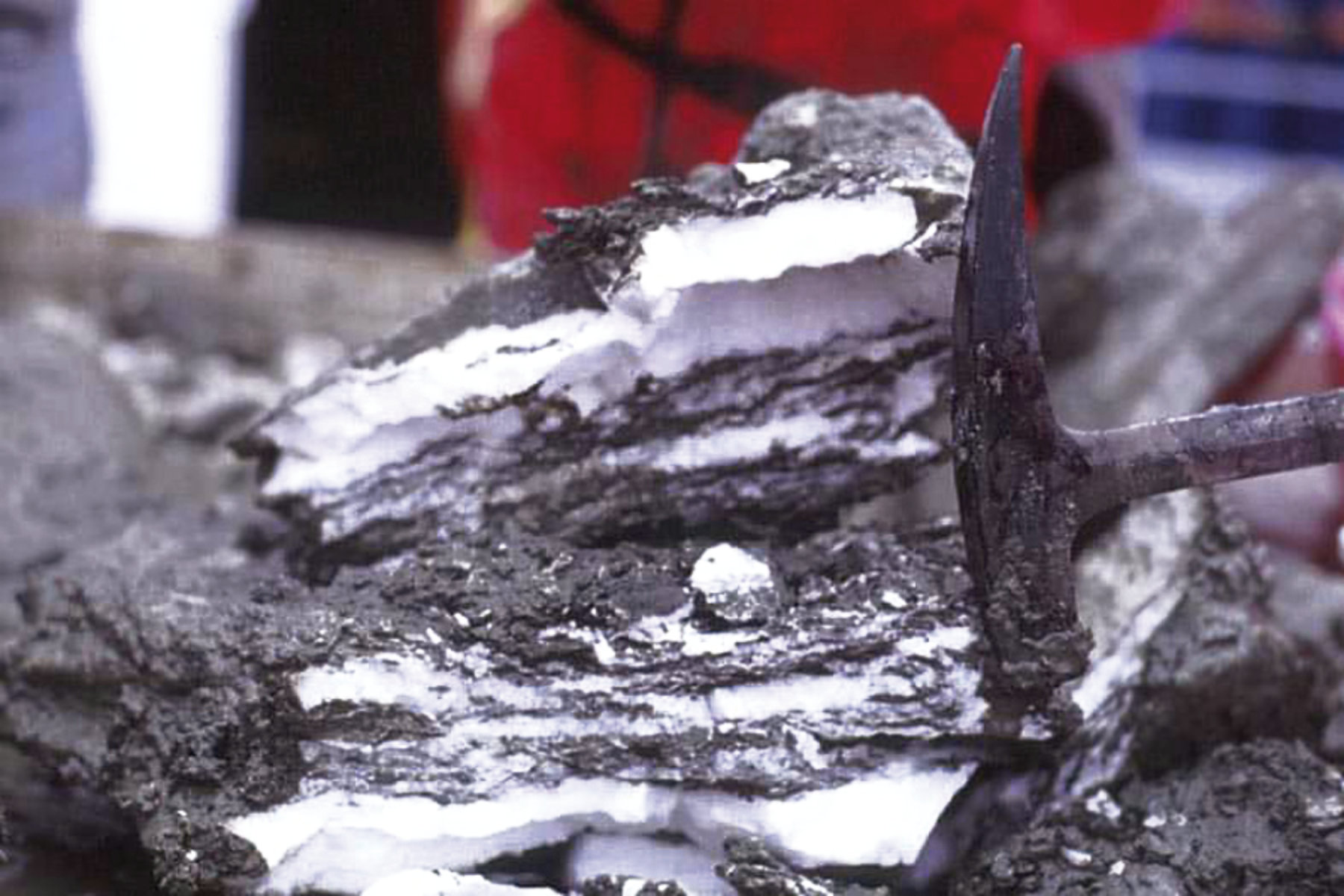
References
[1] Petersen, S., Krätschell, A., Augustin, N., Jamieson, J., Hein, J. R. und Hannington, M. D. (2016) News from the seabed – Geological characteristics and resource potential of deep-sea mineral resources Marine Policy, 70 . pp. 175-187. DOI 10.1016/j.marpol.2016.03.012.
[2] Hasenclever, J., Theissen-Krah, S., Rüpke, L. H., Morgan, J. P., Iyer, K., Petersen, S. und Devey, C. W. (2014) Hybrid shallow on-axis and deep off-axis hydrothermal circulation at fast-spreading ridges Nature, 508 (7497). pp. 508-512. DOI 10.1038/nature13174.
[3] van Doorn, E. (2016) Environmental aspects of the Mining Code: Preserving humankind's common heritage while opening Pardo's box? Marine Policy, 70, 192-197.
[4] Hannington, Mark D., Petersen, Sven und Krätschell, Anna (2017) Subsea mining moves closer to shore Nature Geoscience, 10 (3). pp. 158-159. DOI 10.1038/ngeo2897.
[5] Kretschmer, K., Biastoch, A., Rüpke, L. und Burwicz, E. (2015) Modeling the fate of methane hydrates under global warming Global Biogeochemical Cycles, 29 (5). pp. 610-625. DOI 10.1002/2014GB005011.
[6] Doepke, L. K. und Requate, T. (2014) The economics of exploiting gas hydrates Energy Economics, 42 . pp. 355-364.